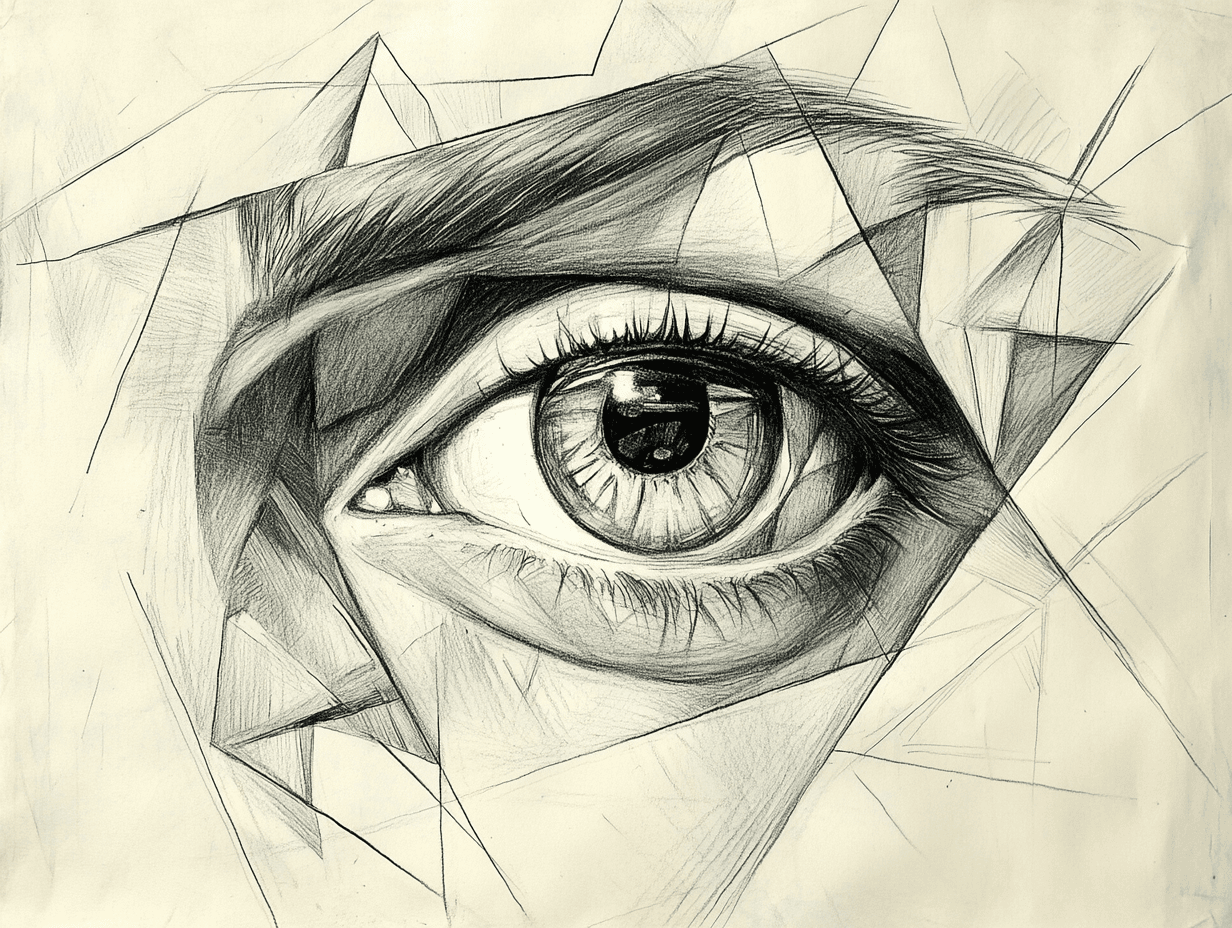
To suppose that the eye with all its inimitable contrivances for adjusting the focus to different distances, for admitting different amounts of light, and for the correction of spherical and chromatic aberration, could have been formed by natural selection, seems, I freely confess, absurd in the highest degree.
On the Origin of Species, Chapter VI, 1859, Charles Darwin
For creatures that have the ability to move1, escaping predation often involves an array of sophisticated sensory machines to detect and evade threats. These biological marvels typically operate by perceiving waves—be they vibrations coursing through the ground or sound waves rippling through the air—or by detecting particles, as in the case of smell. Yet among these sensory apparatuses, one stands out as particularly fascinating: the eye. This remarkable organ captures light which propagates through vacuum and exhibits a dual nature, sometimes behaving as a wave, other times as a particle. Through a complex interplay of physics and biology, the eye transmits crucial information about our environment, serving as a cornerstone of survival and perception.
The visual organ takes very diverse forms in the animal world and often presents an extreme complexity, implementing all the optical principles known to Physics. Even Charles Darwin was perplexed by this level of complexity.
Few organs in the human body correspond so perfectly to our definition of a Machine. Our eye is a system composed of a lens that concentrates light towards a retina equipped with photoreceptors that convert the energy of photons into an electrical signal. This signal feeds into yet another machine process, creating a cascade of information processing.
This feedback loop evokes Ivan Pavlov, whose experiments with dogs demonstrated how visual stimuli—the mere sight of food—could trigger physiological responses like salivation2. Yet, the eye's role in feedback mechanisms extends far beyond this classic example, manifesting in myriad forms across the animal kingdom, from the most rudimentary organisms to the most complex.
In Cybernetics, Wiener illustrates how flight is induced in extremely simple organisms3 that do not have proper eyes: the flatworm exhibits negative phototropism, meaning that it naturally heads toward the darker region of its environment. This is due to a signal sent by two photoreceptors to the muscles of the trunk which, in turn, contract on the darker side, if any. Thus the worm always turns towards the darker side.
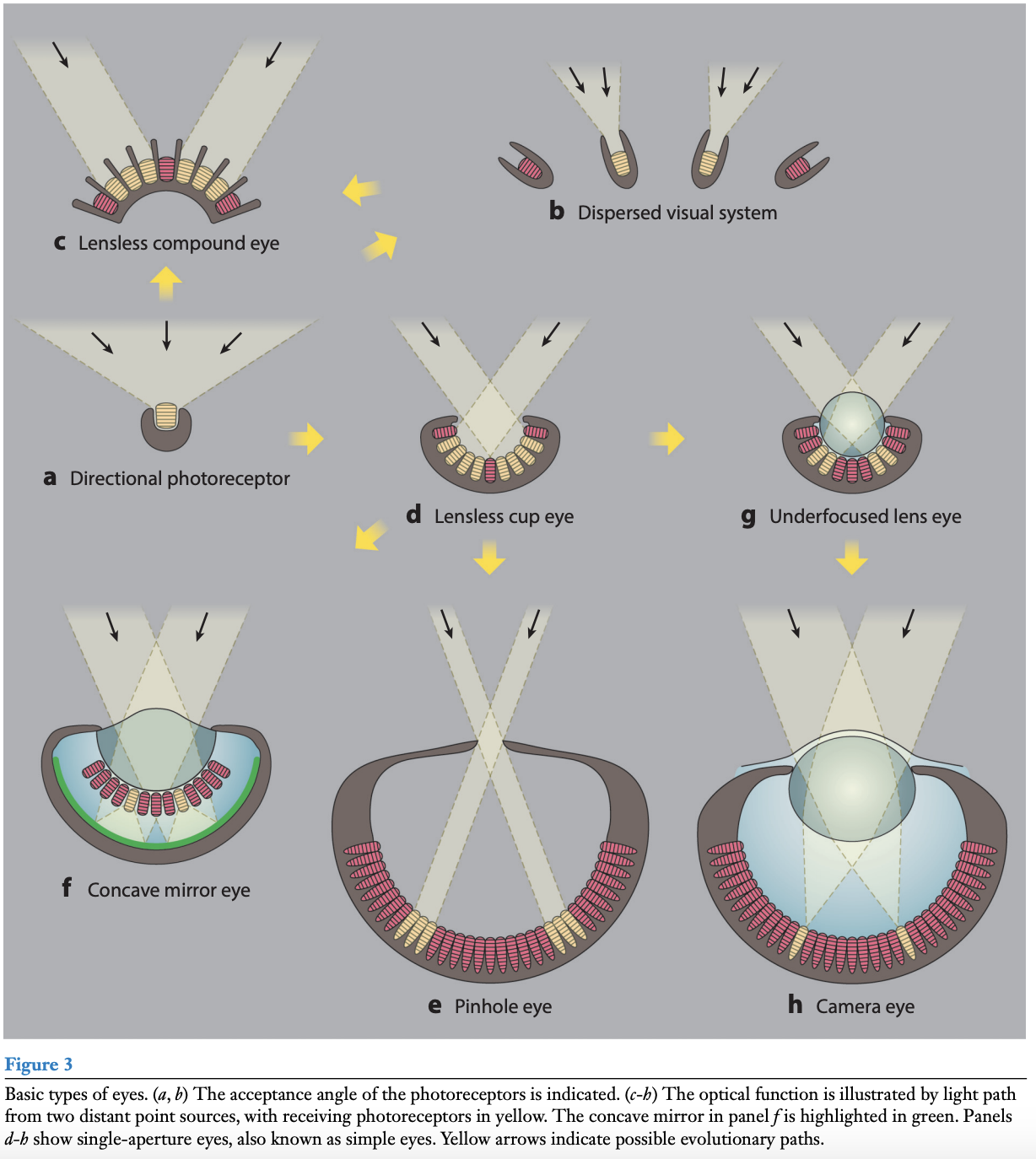
Evolution has built extremely sophisticated machines upstream of the photoreceptors, and their diversity is disconcerting. For example, our eye has only one lens and one retina, while in insects or crustaceans, the eye is divided into a multitude of compartments that all have a retina. Some eyes are convex, while others incorporate internal mirrors, further expanding the range of optical solutions. This remarkable variety, coupled with the puzzling observation of similar eye configurations in distantly related species and divergent designs in close relatives, long confounded attempts to trace a common ancestral origin for vision. Adding to the mystery, even the earliest known fossils from the Cambrian era already showcase relatively complex ocular systems.
Professor Dan-Eric Nilsson of Lunds University in Sweden proposed a key to the mystery by breaking down the evolution of Nature's optical machines into four distinct phases:
- The common denominator of all vision methods is a family of proteins called opsins that bind to molecules capable of absorbing the energy of photons, called chromophores. All opsins have a common ancestor that bound to melatonin, the hormone that regulates the internal clock of organisms. Melatonin is destroyed by light and its absence is what signals to us that it is daytime. The organism must continually produce melatonin to be able to notice its disappearance. Unlike melatonin, chromophores absorb or shed the energy of light without being destroyed so, when the ancestor of opsins mutated to bind to chromophores, organisms got equipped with reusable light sensors. In this first phase of evolution of the visual system, there was no vision per se but simply a perception of light intensity, which could be summarized as a vision of the world with a resolution of 1 pixel.
- In the second phase of evolution, the primitive eye managed to determine the direction of light. This is made possible by a new protective layer with pigments that block light in certain directions.
- The third phase saw the photoreceptors form clusters pointing in slightly different directions. The field of vision widened.
- Finally, in the fourth phase, the eye acquired a lens that concentrates light in a specific location and therefore to obtain a sharper image. In some cases, mirrors play the role of the lens. Lenses or mirrors seemingly appeared independently but convergently during evolution.
Nilsson's simulations showed that even in conservative scenarios, a few hundred thousand generations would allow the transition from the second phase of evolution to the fourth. The eye is therefore an organ that evolves very quickly, and this probably explains the diversity of visual systems across the animal kingdom. No eye is the perfect optical machine, but each visual system is perfectly adapted to a specific use case. What's particularly interesting in the case of this rapid and diverse evolution is that we observe that some species have lost features previously acquired by their ancestors.
Among vertebrates, most birds and reptiles perceive colors thanks to photoreceptor cells in the retina called cones4. There are four types of cones corresponding to the wavelengths of blue, green, red and ultraviolet light. But mammals evolved from nocturnal ancestors that did not require color perception. Nature's tendency for optimization led primitive mammals to abandon two types of cones. As a result, most mammals only perceive a limited range of colors5. Marine mammals eventually lost the cone corresponding to blue, and some whales also gave up on red, relying mainly on rods - the other category of light-sensitive cells that allow night vision. However, primates have re-developed a third type of cone that expands the range of reds and oranges, apparently to better distinguish ripe fruit. Color blindness comes from an abnormality of one of these cones, and conversely, it is estimated that 10% of men and up to 50% of women have a fourth type of cone that allows for even more subtle color perception.
Like many of Nature's machines, the eye reminds us of an important fact: when the energy needed to power the machine is wasted, the machine eventually disappears. The eye is a gluttonous machine because the neurons that carry electrical signals from the photoreceptors must be always ready to activate; the machine is always on standby. This is why animals that evolved to live in environments where there is no light—such as caves or ocean depths—very quickly lost the ocular system that their ancestors had developed.
Our camera eye is an extremely sophisticated machine with a first refractive system, the cornea, upstream of the crystalline lens. An insect would need an eye one meter wide to have the same resolution as us. And as we have seen, we have a finer perception of colors than most mammals. There are many reasons to marvel at our own optical machine. Weiner was, for example, amazed by our ability to understand sketches without colors, shadows or depth3. However, our eye remains so imperfect that it would have been surprising if Humans had been satisfied with it:
- the crystalline lens is the source of many visual disorders, either innate (myopia, hyperopia, astigmatism, etc.) or resulting from aging (presbyopia, cataracts, etc.). As a result, the vast majority of human beings live all or part of their lives with failing vision. And the increase in life expectancy is accompanied by an increase in life expectancy with failing vision.
- Regardless of any failure of our visual organ, randomness in its constitution means that certain perceptions are subjective. For example, the cones form mosaics and the different types of cones are arranged randomly in these mosaics, which implies that the perception of colors can differ from one individual to another, even if they all have the same three types of cones.
- Finally, to top it all off, our eye is organized in such a way that the photoreceptor cells are intertwined or even masked by the network of neurons that transport the electrical signals. As if we were placing a camera behind its cables. The nerve fibers meet at the back of the eye where they pass through a hole to reach the brain. This hole is what explains the blind spot (papilla) in our visual field.
Just as Humans sought to enhance Nature's reproductive machine and immune system, they predictably turned their attention to augmenting their suboptimal optical machine. However, vision proved to be an exceptionally challenging frontier. The intricate link between the science of vision and the science of light long eluded scientists, presenting a seemingly insurmountable obstacle. This connection between vision and light is so fundamental that unraveling the mysteries of the optical machine necessitated uncovering some of the most profound laws of our universe. We'll cover this in the next post of this series.
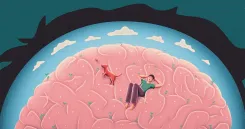
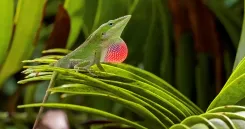
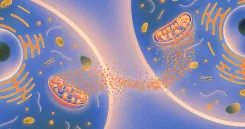